We’d found an old Bridgeport mill, dragged it home and placed it in a convenient corner of our garage. Now there was one last step before we could start machining: Get it running.
As with any used car, plan on going through a used mill after buying it. Ours was missing some hardware.
Fortunately we live in modern times, so replacements were just a few clicks away. We ended up spending about $500 and a month finding, ordering, making and installing the necessary parts–mainly a new leadscrew (the threaded rod that moves our X-axis) and powerfeed (basically a motor).
Getting Power
Machine assembled, we needed to power it–and that’s where things got complicated.
Like most industrial machinery, our Bridgeport came with a three-phase electric motor. No matter what sort of plug we attached, it wouldn’t run from the single-phase power supply in our garage.
What the heck is a three-phase power supply? In the most basic terms, it’s a completely different type of power found only in commercial facilities, as it’s more efficient at driving big electric motors like the one on our Bridgeport.
Getting our power company to supply three-phase power to our residential address was somewhere between insanely expensive and impossible, so we needed to somehow convert this three-phase machine to run on single-phase power.
For years, the easiest way to do this was with a rotary phase converter. These big, expensive machines–about $500 for one appropriately sized–turn single-phase power into three-phase power.
But this is 2022, and there’s a more affordable, smaller, simpler way to run a three-phase motor: a variable frequency drive. Your electrician can tell you more, but basically a VFD costs less than $100, is about the size of a burrito, and can easily run three-phase motors on single-phase loads.
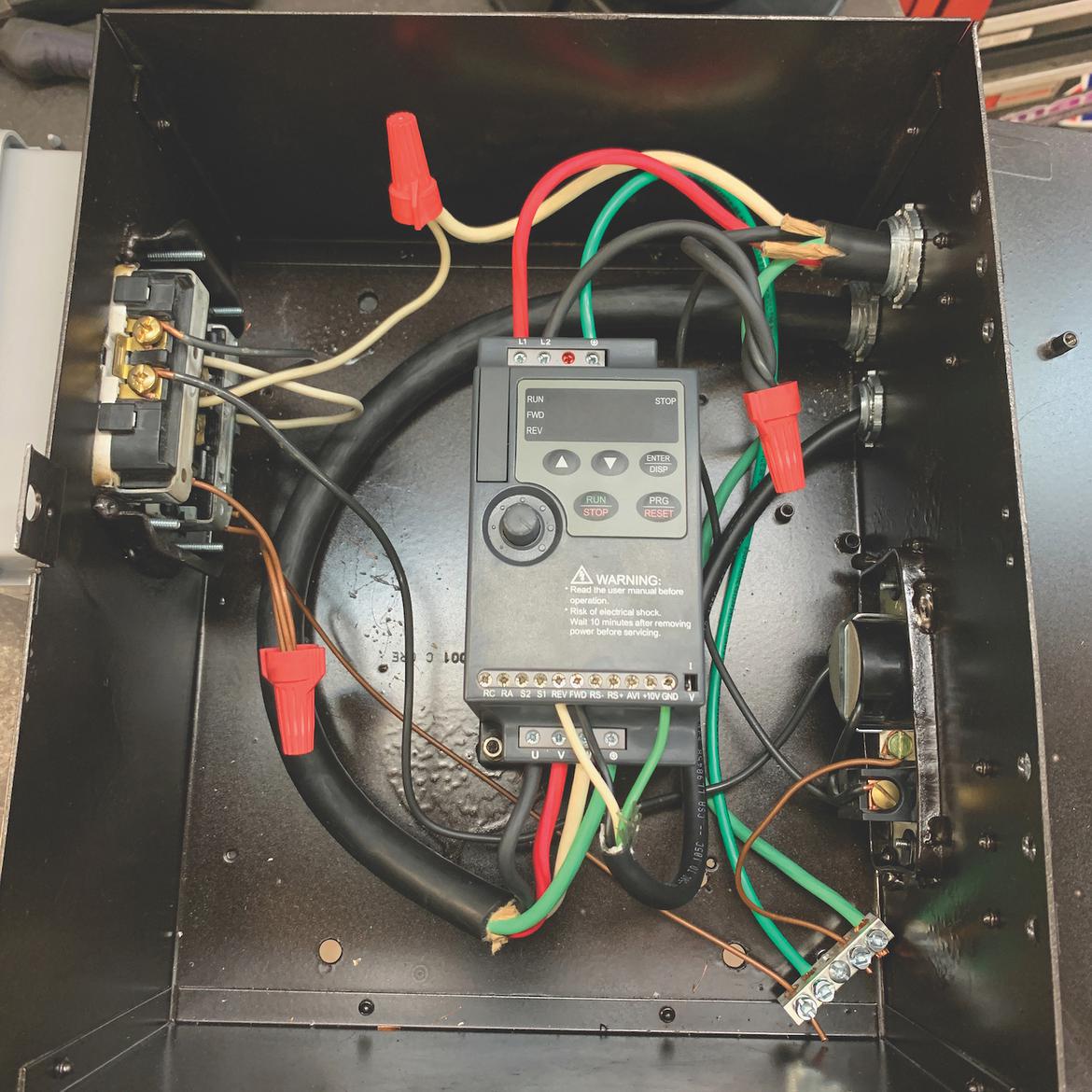
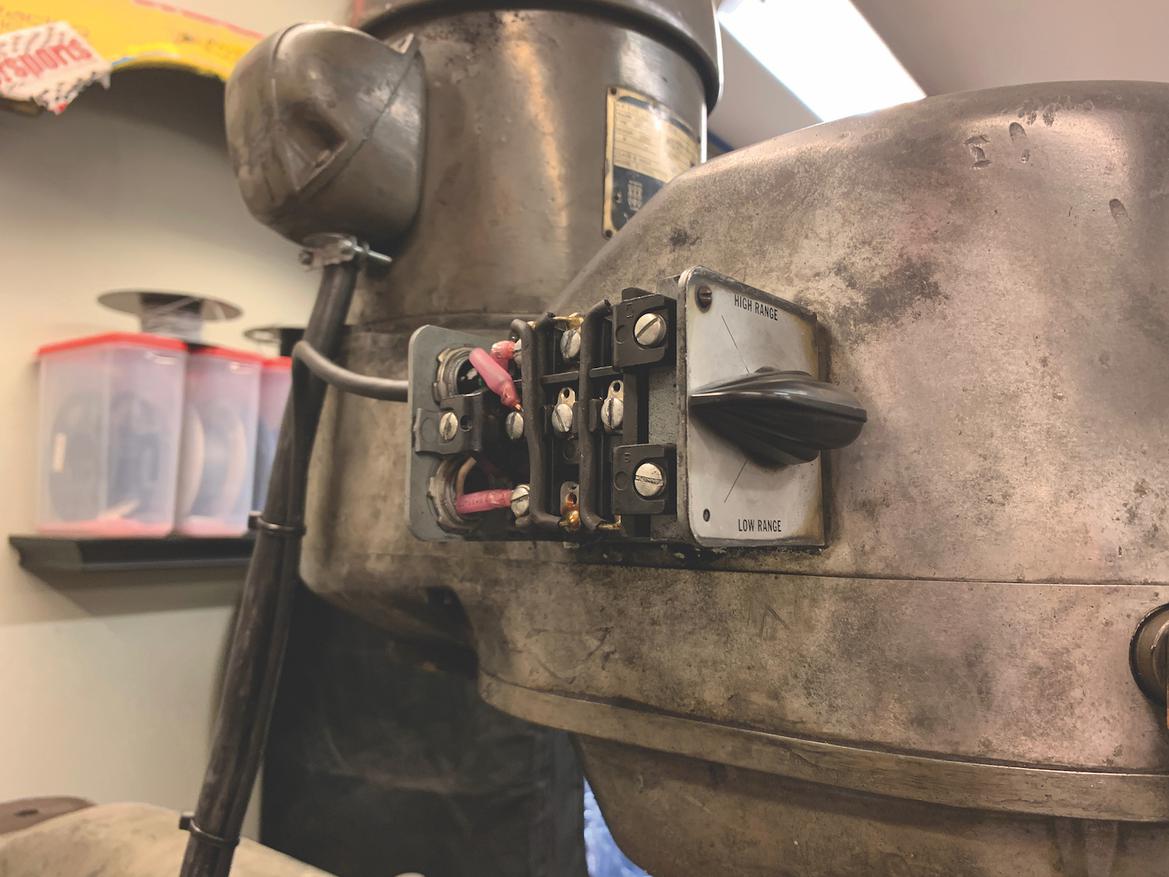
Our Bridgeport, like a lot of heavier industrial equipment, uses three-phase power, something you’re not likely to have in your garage. To power the mill in our home shop, we wired in a variable frequency drive.
We ordered one online, then built an enclosure for it on the side of our machine using parts from a local electrical surplus store. Just like that, our Bridgeport could plug into the standard welding outlet in our garage. We even wired in the OEM motor switch to control the VFD so our machine still looks and runs just like any other Bridgeport.
VFDs have one other trick up their sleeve: They can infinitely vary the speed of the motor they’re driving, which is great if your mill doesn’t have a variable speed head like ours. We didn’t need the speed control, so we set our VFD to always run at 60 hertz, the standard for the U.S.
Can You Read Me Now?
We now owned a Bridgeport with working axes and a running motor, but after using the mill for few simple projects, we realized we just couldn’t make accurate parts with it. After double-checking our setup, we figured out the problem: We simply weren’t good enough with the dials on our Bridgeport.
Each handle has a dial graduated in thousands of an inch, and turning a handle 10 thousandths of an inch theoretically means the tool will move 10 thousandths.
So why couldn’t we make accurate parts? Simple: compounding error, the machinist’s only natural predator. Every time we turn a knob, it’s not exactly perfect–that would be impossible. So a 0.010-inch move might actually be 0.011 or 0.009 inch.
This gets worse when you change directions, as every leadscrew has backlash. If an operation has 20 movements, the errors quickly add up.
These problems are minor when you’re making big, simple parts–who cares if something is 3.001 or 2.999 inches long?–but they’re significant when it comes to small components that need to fit together.
Oh, and using the dials is slow, too. Every move requires knowing where the tool sits and where it needs to be so you can add or subtract the right motion. Keeping track of where the tool is across each move requires a calculator and scratch paper, which takes time and offers more opportunity for error.
There are two ways to solve these problems. The first: Become a machining savant who can spin each knob perfectly, correct for error and backlash in real time, and track the tool without scratch paper. That’s probably how people did this in the 1930s, but we don’t have the time or patience, and perfecting our technique with knobs will never be as accurate as the other option: Install a digital readout, commonly abbreviated as DRO.
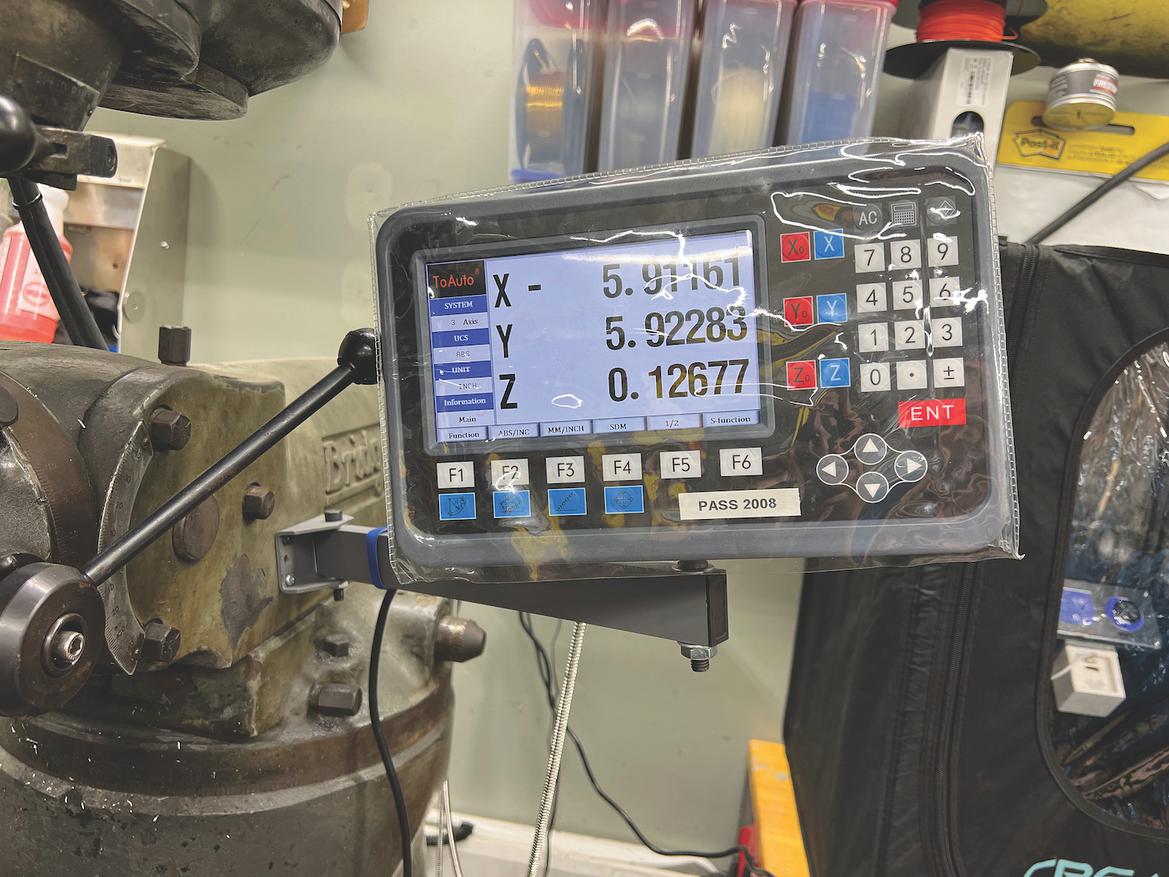
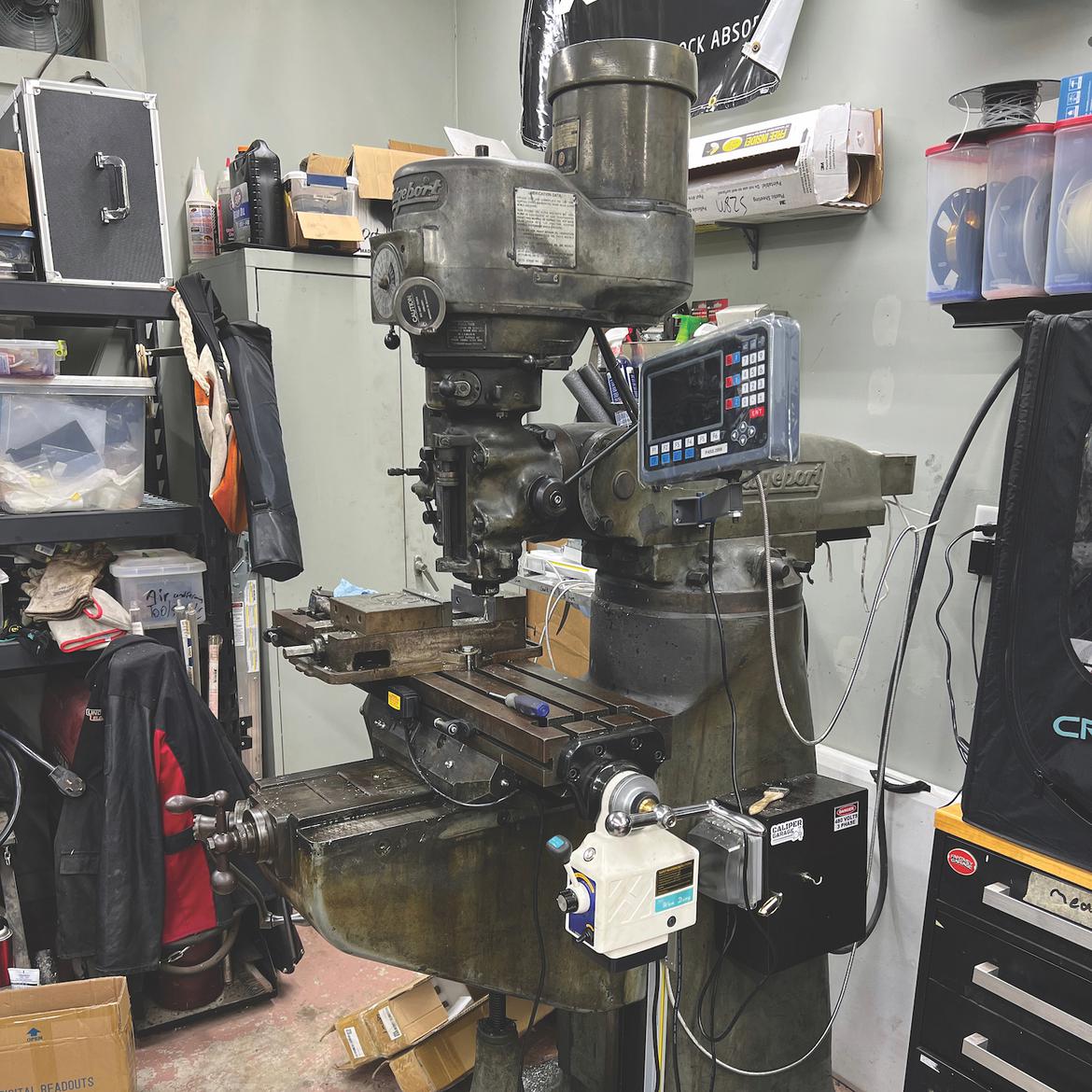
More modern technology: Instead of turning knobs to make cuts, we installed a digital readout.
DROs are awesome because they track the position of the axis, not the handles or screws. In other words, rather than measuring the input and inferring the output–like those scales on each knob–they just track the output. This is more accurate and means measurement errors don’t compound over time. Plus, DROs display this information on an easy-to-digest display, which also incorporates a calculator and some built-in formulas to compute more complex geometry.
Okay, let’s go DRO shopping. You can buy a name-brand DRO for about $2000 or an off-brand one for as little as $200. Prices vary based on the quality of the display, the quality of the instruments actually measuring each axis, the mounting hardware included, the number of axes, and the post-sales support.
We chose a generic, imported LCD display, pairing it with generic glass scales. We measured our machine’s x, y and knee (z) travel, then specced the glass scales to fit.
DROs can measure tool position via glass scales, like our setup, or via magnetic scales. These are less breakable but also seem to cost three to five times more. Glass scales work fine, theoretically, as long as you can keep a strip of glass clean and intact next to a machine that literally shakes the ground when it’s turned on. Fingers crossed.
All told, this DRO system cost $391. We saved lots of money by making our own mounts for everything, choosing an off-brand, and selecting the more fragile glass scale design. As little as we’ll use this machine compared to a production shop, we didn’t feel the more durable scales were worth the price increase. We’ll just have to make sure to keep these away from impacts and coolant. The LCD display was admittedly a bit of a splurge, but the interface should be much simpler to understand than an eight-digit LED display with mediocre instructions.
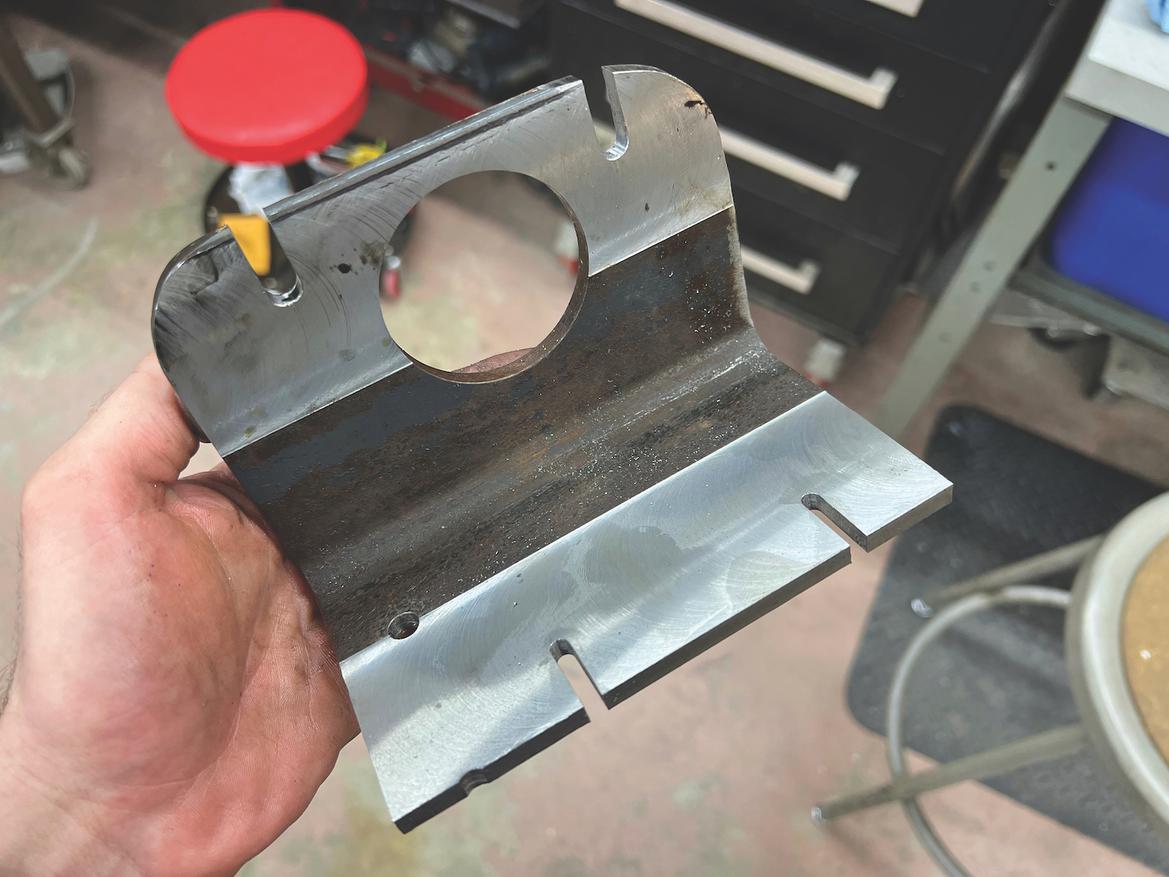
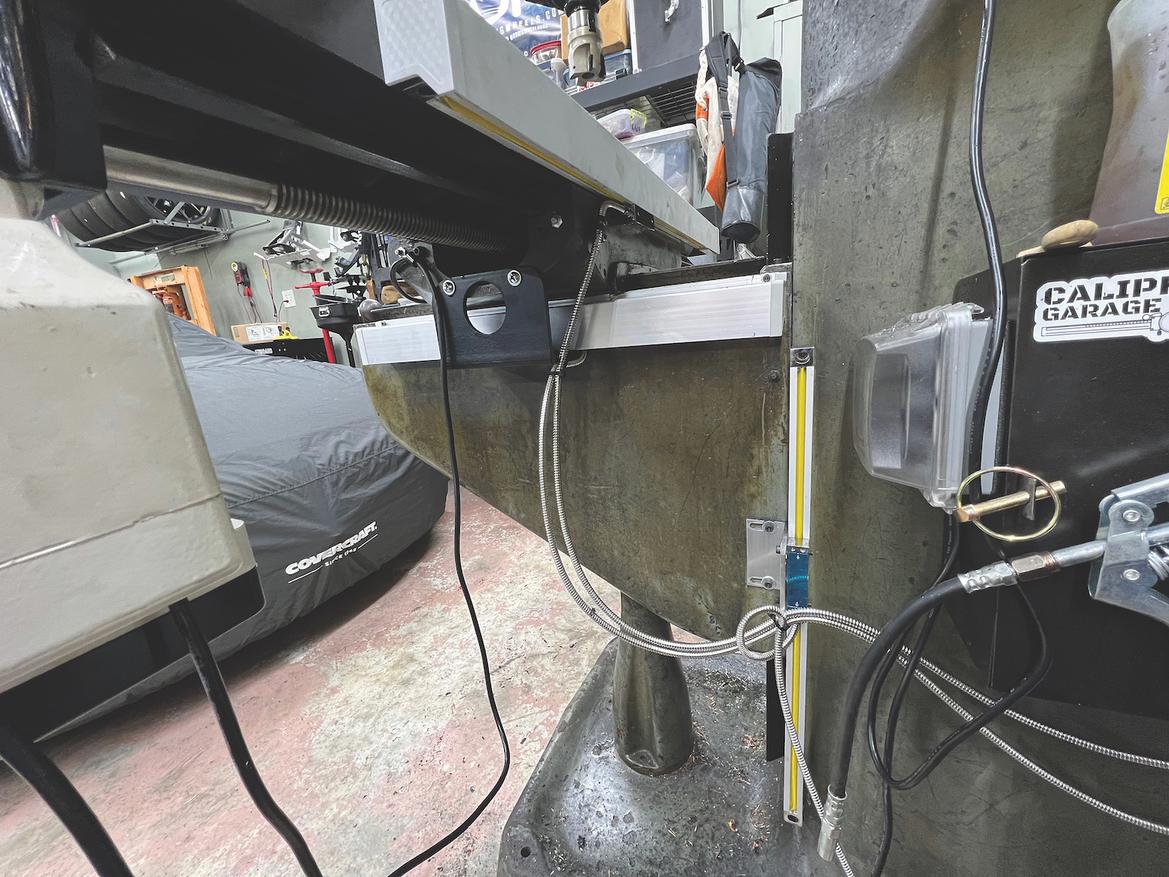
We had to make some brackets to attach the required glass scales, but for less than $400 we improved our mill’s accuracy.
Installation took a few evenings and mostly involved drilling and tapping lots of holes. In fact, we only needed to make one part, a mount for our machine’s y-axis scale head. Finally, we could accurately move the machine along each of its three axes. It was time to make some parts.
What’s Next?
You may not have realized it, but there’s been an elephant in the corner for this entire story: tooling, or the odds and ends we pair with our mill to actually make parts.
Common wisdom says buying tooling will dwarf our initial investment in the machine itself, meaning we’ll spend at least $3000 just on accessories. But is that true, and does it have to be? We’ll find out in the next installment of this series, when we buy a vise, rotary table and everything else needed to make parts with our new machine.